Our Research
Advances in research through focus and expertise.
Our Research
Advances in research through focus and expertise.
Diffuse Intrinsic Pontine Glioma (DIPG)
The Rutka Lab is developing new ways to treat this universally fatal paediatric brain tumour.
Unlike some tumour types, DIPG cannot be surgically removed.
DIPG is inoperable because of its location within the brainstem. The brainstem (outlined in yellow) controls vital functions like breathing, heart rate and blood pressure. The DIPG tumour is highlighted in pink.
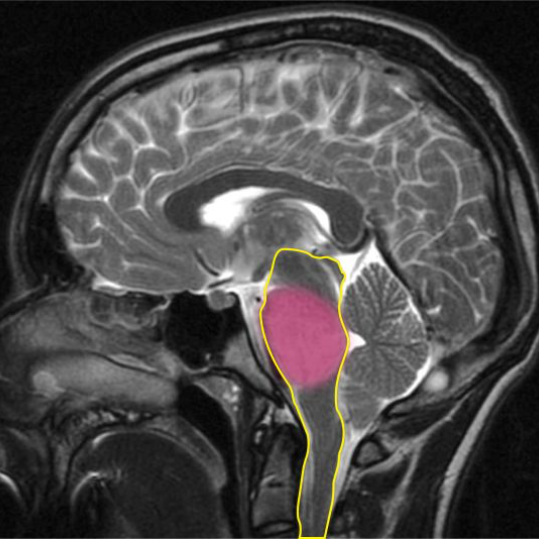
The Rutka Lab is developing new ways to treat this universally fatal paediatric brain tumour.
Unlike some tumour types, DIPG cannot be surgically removed.
DIPG is inoperable because of its location within the brainstem. The brainstem (outlined in yellow) controls vital functions like breathing, heart rate and blood pressure. The DIPG tumour is highlighted in pink.
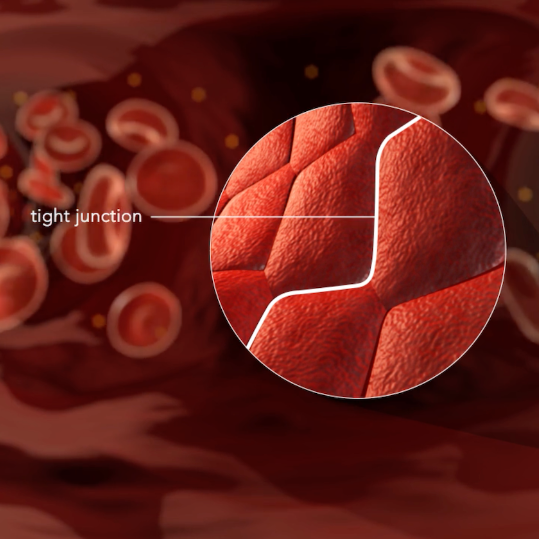
The blood-brain barrier presents a unique problem.
Like a security gate, the blood-brain barrier (BBB) protects the brain – stopping toxins, viruses and other harmful compounds from leaving the bloodstream and entering the brain.
In the same way, the BBB prevents helpful chemotherapies from reaching tumour targets.
In fact, there have been hundreds of clinical trials for DIPG using drugs that are predicted to work in patients, but because the BBB blocks these new drugs from reaching the tumour, the clinical trials inevitably fail.
The blood-brain barrier presents a unique problem.
Like a security gate, the blood-brain barrier (BBB) protects the brain – stopping toxins, viruses and other harmful compounds from leaving the bloodstream and entering the brain.
In the same way, the BBB prevents helpful chemotherapies from reaching tumour targets.
In fact, there have been hundreds of clinical trials for DIPG using drugs that are predicted to work in patients, but because the BBB blocks these new drugs from reaching the tumour, the clinical trials inevitably fail.
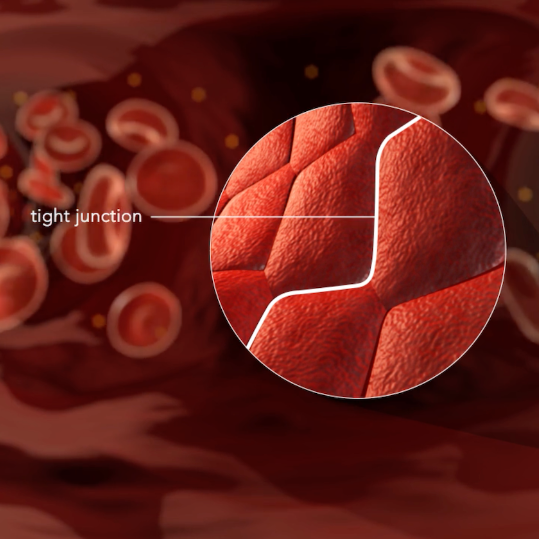
The blood-brain barrier presents a unique problem.
Like a security gate, the blood-brain barrier (BBB) protects the brain – stopping toxins, viruses and other harmful compounds from leaving the bloodstream and entering the brain.
In the same way, the BBB prevents helpful chemotherapies from reaching tumour targets.
In fact, there have been hundreds of clinical trials for DIPG using drugs that are predicted to work in patients, but because the BBB blocks these new drugs from reaching the tumour, the clinical trials inevitably fail.
To overcome this obstacle, we collaborated with Dr. Kullervo Hynynen and his biophysics lab at Sunnybrook Hospital.
We developed a way to test drug delivery by temporarily opening the BBB using ultrasound waves.
This gives us a four-hour window to administer chemotherapy directly to the tumour. MRI machines are used to focus the ultrasound to ensure none of the surrounding healthy tissue is affected. Photo credit: Sunnybrook Health Sciences Centre.
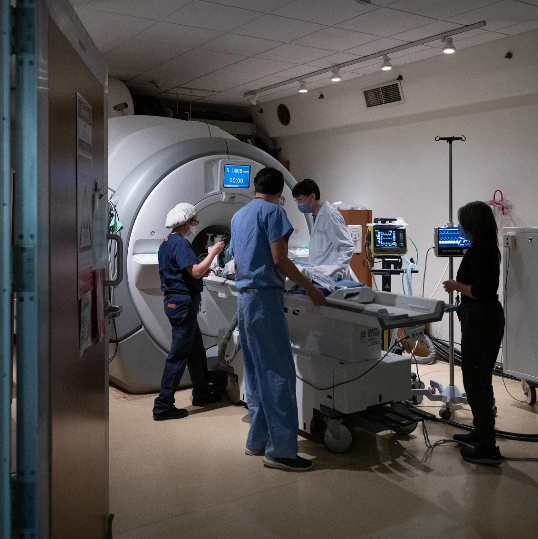
To overcome this obstacle, we collaborated with Dr. Kullervo Hynynen and his biophysics lab at Sunnybrook Hospital.
We developed a way to test drug delivery by temporarily opening the BBB using ultrasound waves.
This gives us a four-hour window to administer chemotherapy directly to the tumour. MRI machines are used to focus the ultrasound to ensure none of the surrounding healthy tissue is affected. Photo credit: Sunnybrook Health Sciences Centre.
“Nothing in life is to be feared, it is only to be understood. Now is the time to understand more, so that we may fear less.”
– Marie Curie
How did we get here? Background research was conducted in mouse models.
The brains of mice and humans are remarkably evolutionarily conserved, which means the cell types making up the brain and its structural architecture are similar. Consequently, mouse models that develop DIPG tumours within the mouse brainstem can be utilized experimentally. We set out to test the effectiveness of targeted chemotherapy when paired with MRI-guided focused ultrasound (MRgFUS) in mouse models of DIPG.
DIPG mouse models exhibit the same molecular markers as clinical DIPGs. Immunohistochemistry on SU-DIPG-17 xenografts (mice with human tumours) revealed expression patterns for 4 common DIPG molecular markers (Ki-67, Nestin, p53, H3K27me3) that closely resembled those seen in clinical patient samples.
How did we get here? Background research was conducted in mouse models.
The brains of mice and humans are remarkably evolutionarily conserved, which means the cell types making up the brain and its structural architecture are similar. Consequently, mouse models that develop DIPG tumours within the mouse brainstem can be utilized experimentally. We set out to test the effectiveness of targeted chemotherapy when paired with MRI-guided focused ultrasound (MRgFUS) in mouse models of DIPG.
DIPG mouse models exhibit the same molecular markers as clinical DIPGs. Immunohistochemistry on SU-DIPG-17 xenografts (mice with human tumours) revealed expression patterns for 4 common DIPG molecular markers (Ki-67, Nestin, p53, H3K27me3) that closely resembled those seen in clinical patient samples.
Enhancement of BBB permeability via MRgFUS resulted in increased doxorubicin uptake in SU-DIPG-17 tumours. A) A targeting grid (right) was mapped to cover the entire tumour region prior to ultrasound treatment. B) The tumour area targeted on the T2 weighted image was treated with ultrasound and the contrast agent Gadolinium was observed in the same area in post treatment images (right) suggesting successful BBB permeability and uptake of circulating contrast agent.
Choosing the right chemotherapeutic
Doxorubicin was selected from a drug screen consisting of conventional chemotherapeutics tested on patient-derived cell lines. Doxorubicin was the ideal choice because it not only demonstrated efficacy on DIPG cell lines, but also because it normally doesn’t cross the BBB – shielding the normal brain tissue and limiting potential side effects.
Mice that develop DIPG tumours demonstrated a diffusely infiltrative tumour growth pattern similar to human DIPG. In our study, SU-DIPG-17 xenografts were more representative of human DIPG with an intact BBB.
The importance of choosing the right chemotherapeutic
Doxorubicin was selected from a drug screen consisting of conventional chemotherapeutics tested on patient-derived cell lines. Doxorubicin was the ideal choice because it not only demonstrated efficacy on DIPG cell lines, but also because it normally doesn’t cross the BBB – shielding the normal brain tissue and limiting potential side effects.
Mice that develop DIPG tumours demonstrated a diffusely infiltrative tumour growth pattern similar to human DIPG. In our study, SU-DIPG-17 xenografts were more representative of human DIPG with an intact BBB.
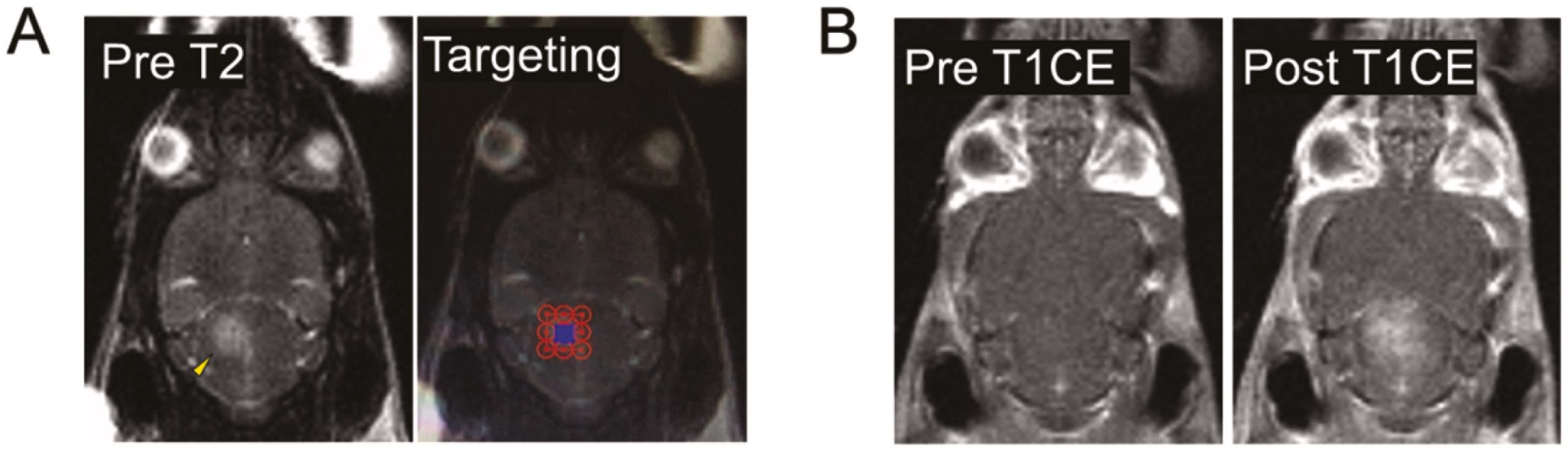
Enhancement of BBB permeability via MRgFUS resulted in increased doxorubicin uptake in SU-DIPG-17 tumours. A) A targeting grid (right) was mapped to cover the entire tumour region prior to ultrasound treatment. B) The tumour area targeted on the T2 weighted image was treated with ultrasound and the contrast agent Gadolinium was observed in the same area in post treatment images (right) suggesting successful BBB permeability and uptake of circulating contrast agent.
Choosing the right chemotherapeutic
Doxorubicin was selected from a drug screen consisting of conventional chemotherapeutics tested on patient-derived cell lines. Doxorubicin was the ideal choice because it not only demonstrated efficacy on DIPG cell lines, but also because it normally doesn’t cross the BBB – shielding the normal brain tissue and limiting potential side effects.
Mice that develop DIPG tumours demonstrated a diffusely infiltrative tumour growth pattern similar to human DIPG. In our study, SU-DIPG-17 xenografts were more representative of human DIPG with an intact BBB.
Enhancement of BBB permeability via MRgFUS resulted in increased doxorubicin uptake in SU-DIPG-17 tumours. A) A targeting grid (right) was mapped to cover the entire tumour region prior to ultrasound treatment. B) The tumour area targeted on the T2 weighted image was treated with ultrasound and the contrast agent Gadolinium was observed in the same area in post treatment images (right) suggesting successful BBB permeability and uptake of circulating contrast agent.
Testing the results of our MRgFUS experiment
Following IV administration of doxorubicin, MRgFUS-treated animals exhibited a 4-fold higher concentration of the chemotherapy drug within the SU-DIPG-17 brainstem tumours compared to controls not exposed to ultrasound.
Testing the results of our MRgFUS experiment
Following IV administration of doxorubicin, MRgFUS-treated animals exhibited a 4-fold higher concentration of the chemotherapy drug within the SU-DIPG-17 brainstem tumours compared to controls not exposed to ultrasound.
An exciting next step – applying our research to the patient population
The successes in our studies has directly resulted in the opening of a world-first clinical trial to test enhanced drug delivery using focused ultrasound in patients with DIPG. The trial is now Health Canada approved and the very first patient enrolled in the trial received ultrasound treatment in January 2023! A huge landmark!
Currently, our lab is extending our focus to finding the right chemotherapy drugs for each patient (called targeted therapy) that will work synergistically to shrink and hopefully eliminate the tumour.
An exciting next step – applying our research to the patient population
The successes in our studies has directly resulted in the opening of a world-first clinical trial to test enhanced drug delivery using focused ultrasound in patients with DIPG. The trial is now Health Canada approved and the very first patient enrolled in the trial received ultrasound treatment in January 2023! A huge landmark!
Currently, our lab is extending our focus to finding the right chemotherapy drugs for each patient (called targeted therapy) that will work synergistically to shrink and hopefully eliminate the tumour.
Glioblastoma
Malignant gliomas are highly aggressive cancers.
Despite surgery, chemotherapy, and radiation therapy, survival expectancy is less than 2 years.
Over the past 30 years, limited progress has been made in treating this aggressive cancer despite our increased understanding of the molecular genetics and basic biology of these tumours. New treatment strategies are desperately needed. The cerebrum (outlined in yellow) is responsible for sending and receiving signals to and from the body. A typical tumour is highlighted in pink.
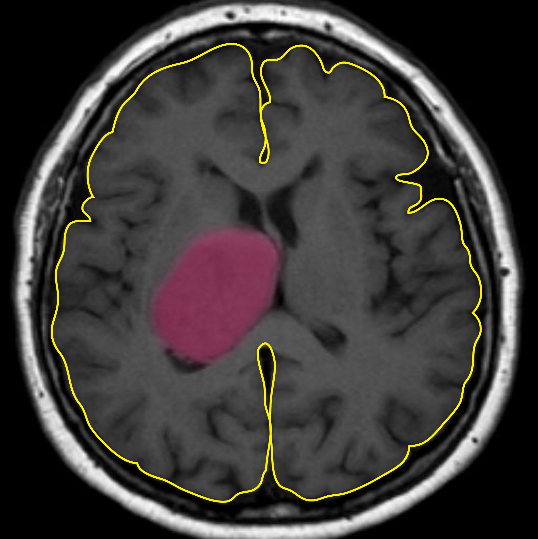
Malignant gliomas are highly aggressive cancers.
Despite surgery, chemotherapy, and radiation therapy, survival expectancy is less than 2 years.
Over the past 30 years, limited progress has been made in treating this aggressive cancer despite our increased understanding of the molecular genetics and basic biology of these tumours. New treatment strategies are desperately needed. The cerebrum (outlined in yellow) is responsible for sending and receiving signals to and from the body. A typical tumour is highlighted in pink.
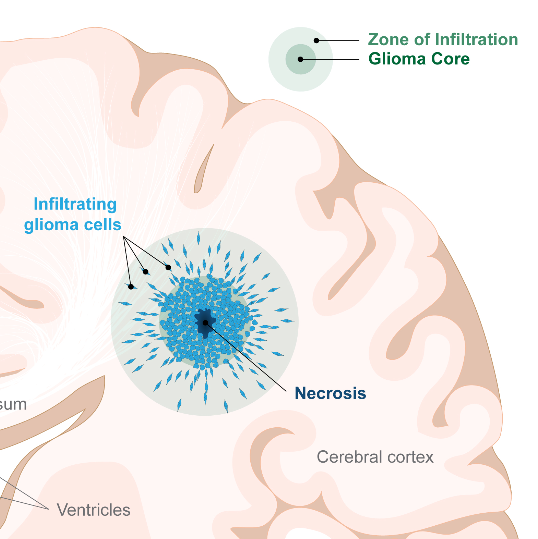
Malignant gliomas are comprised of two zones/types of cells.
There is a central core of cancer cells which is rapidly dividing to increase tumour mass. Cells within the core have evolved ways to resist chemotherapy and radiation, threatening their effectiveness.
There are also highly invasive cells which are not rapidly dividing and can migrate a considerable distance away from the central core. These cells tend to reside in the periphery of the tumour, and are what we refer to as the “zone of infiltration” (ZOI). These invading glioma cells are somewhat protected by an intact blood brain barrier which prevents chemotherapy from effectively reaching them.
Malignant gliomas are comprised of two zones/types of cells.
There is a central core of cancer cells which is rapidly dividing to increase tumour mass. Cells within the core have evolved ways to resist chemotherapy and radiation, threatening their effectiveness.
There are also highly invasive cells which are not rapidly dividing and can migrate a considerable distance away from the central core. These cells tend to reside in the periphery of the tumour, and are what we refer to as the “zone of infiltration” (ZOI). These invading glioma cells are somewhat protected by an intact blood brain barrier which prevents chemotherapy from effectively reaching them.
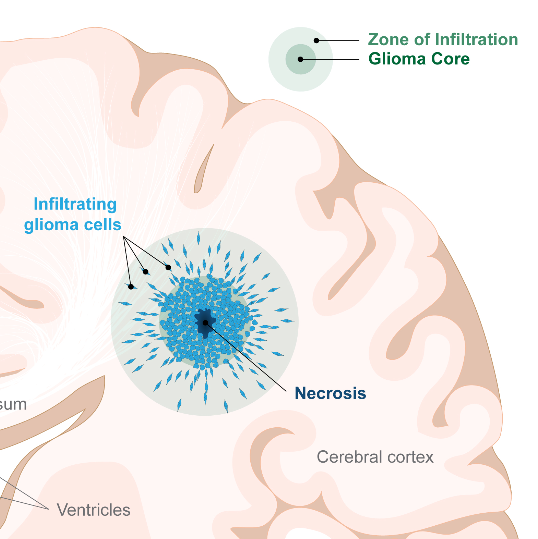
Malignant gliomas are comprised of two zones/types of cells.
There is a central core of cancer cells which is rapidly dividing to increase tumour mass. Cells within the core have evolved ways to resist chemotherapy and radiation, threatening their effectiveness.
There are also highly invasive cells which are not rapidly dividing and can migrate a considerable distance away from the central core. These cells tend to reside in the periphery of the tumour, and are what we refer to as the “zone of infiltration” (ZOI). These invading glioma cells are somewhat protected by an intact blood brain barrier which prevents chemotherapy from effectively reaching them.
Our lab is developing novel therapies to treat the rapidly dividing cells in the glioma core.
One approach is to focus on metabolically reprogramming the core cells.
This reprogramming acts to reduce their proliferation, and to inhibit glioma cells from following pro-survival pathways in response to cellular stresses such as low oxygen levels, chemotherapy, and radiation therapy.
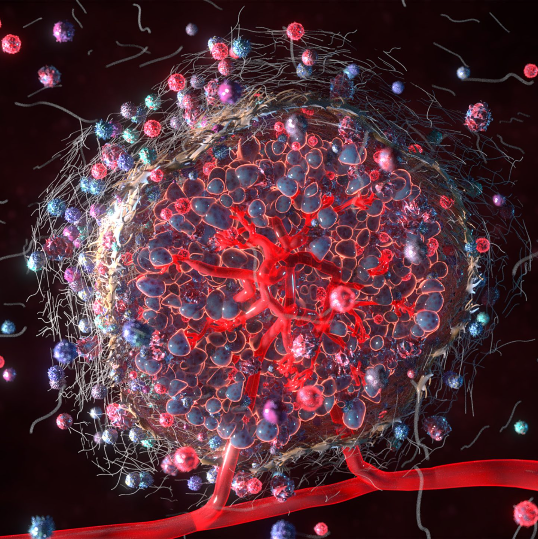
Our lab is developing novel therapies to treat the rapidly dividing cells in the glioma core.
One approach is to focus on metabolically reprogramming the core cells.
This reprogramming acts to reduce their proliferation, and to inhibit glioma cells from following pro-survival pathways in response to cellular stresses such as low oxygen levels, chemotherapy, and radiation therapy.
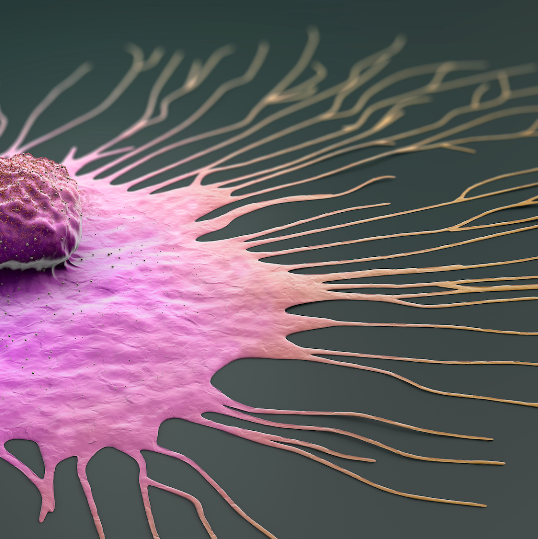
Targeting invading glioma cells is just as important.
How do we do it?
We target invading glioma cells using novel inhibitors of cell invasion, and MRI-guided focused ultrasound to break down the BBB around the tumour core and facilitate contact of inhibitors with the invading cells.
We anticipate that our approach of simultaneous precision targeting of the glioma core and zone of infiltration with potent drugs and advanced delivery strategies will provide therapeutic and survival benefit to patients suffering from this lethal cancer.
Targeting invading glioma cells is just as important.
How do we do it?
We target invading glioma cells using novel inhibitors of cell invasion, and MRI-guided focused ultrasound to break down the BBB around the tumour core and facilitate contact of inhibitors with the invading cells.
We anticipate that our approach of simultaneous precision targeting of the glioma core and zone of infiltration with potent drugs and advanced delivery strategies will provide therapeutic and survival benefit to patients suffering from this lethal cancer.
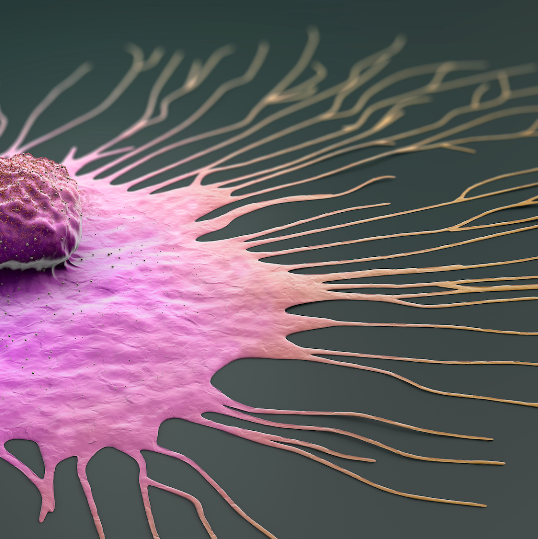
Targeting invading glioma cells is just as important.
How do we do it?
We target invading glioma cells using novel inhibitors of cell invasion, and MRI-guided focused ultrasound to break down the BBB around the tumour core and facilitate contact of inhibitors with the invading cells.
We anticipate that our approach of simultaneous precision targeting of the glioma core and zone of infiltration with potent drugs and advanced delivery strategies will provide therapeutic and survival benefit to patients suffering from this lethal cancer.
Additional Strategies
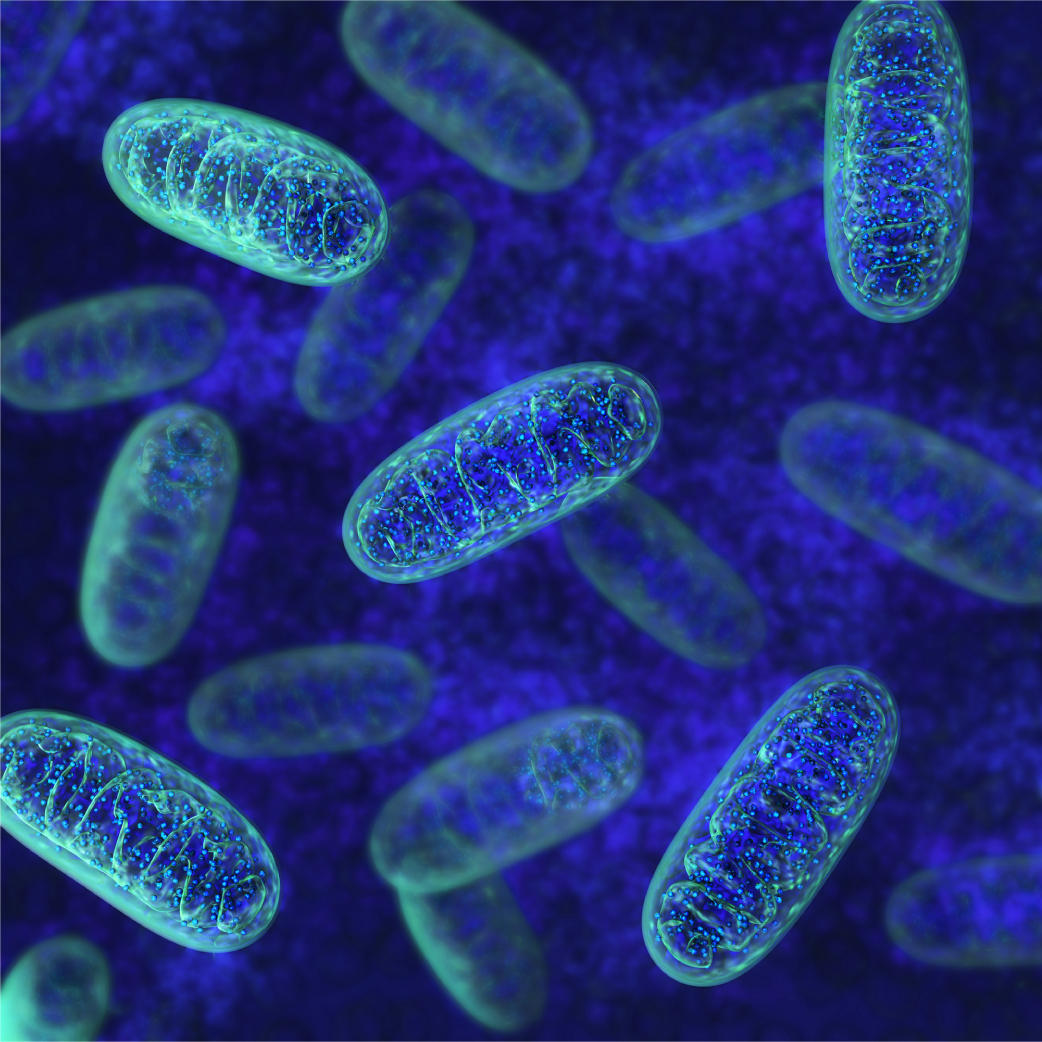
Mitochondria: A new mechanism underlying chemotherapy resistance?
Part of the reason glioblastoma is so difficult to treat is due to its rapid ability to adapt and overcome the effects of chemotherapy and radiation, often within months. We now know that mitochondria, the energy machinery of the cell, plays a role in how glioblastoma responds to chemotherapy and helps these tumours recur after treatment. We have discovered a new mechanism of chemotherapy resistance within the mitochondria that has the potential to be exploited to make cancer cells more sensitive to existing therapies.
Mitochondria: A new mechanism underlying chemotherapy resistance?
Part of the reason glioblastoma is so difficult to treat is due to its rapid ability to adapt and overcome the effects of chemotherapy and radiation, often within months. We now know that mitochondria, the energy machinery of the cell, plays a role in how glioblastoma responds to chemotherapy and helps these tumours recur after treatment. We have discovered a new mechanism of chemotherapy resistance within the mitochondria that has the potential to be exploited to make cancer cells more sensitive to existing therapies.
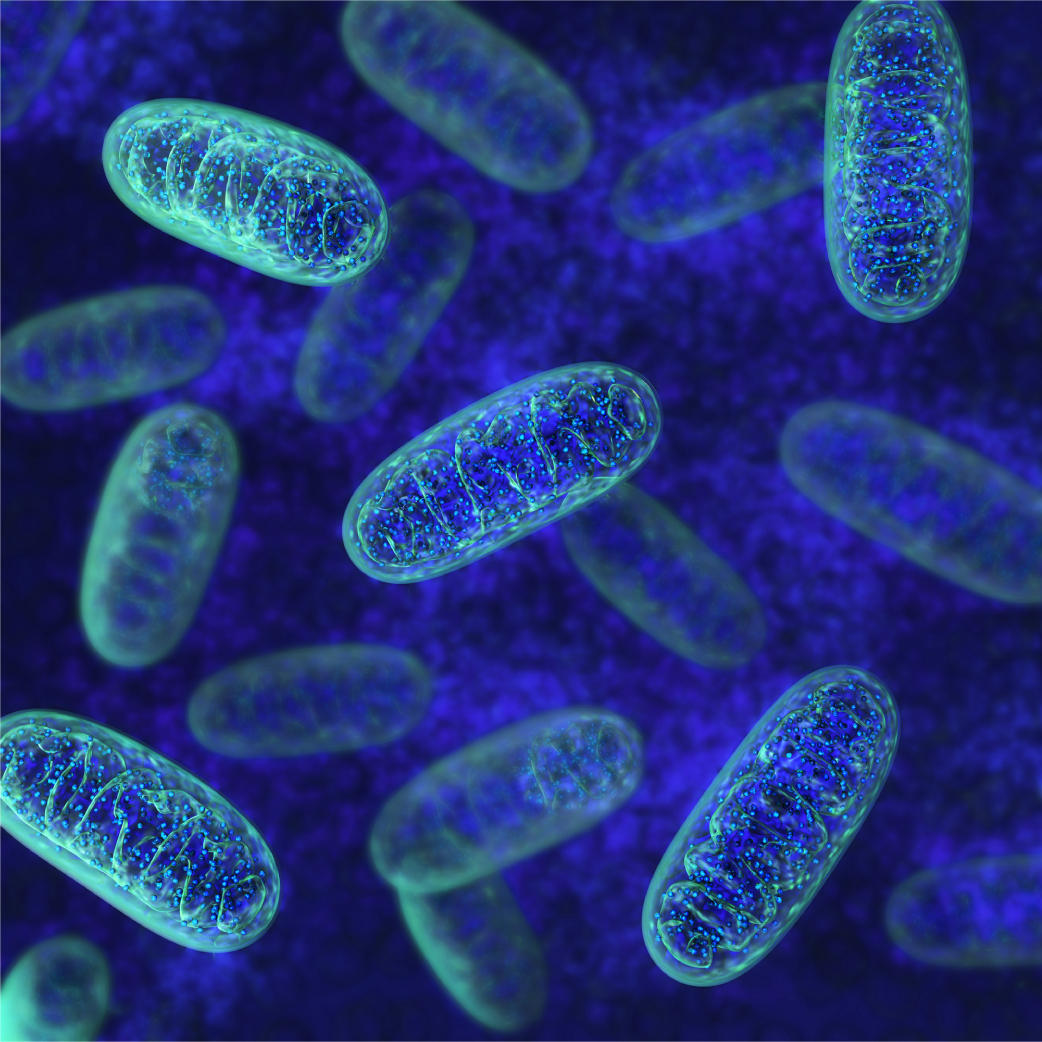
Mitochondria: A new mechanism underlying chemotherapy resistance?
Part of the reason glioblastoma is so difficult to treat is due to its rapid ability to adapt and overcome the effects of chemotherapy and radiation, often within months. We now know that mitochondria, the energy machinery of the cell, plays a role in how glioblastoma responds to chemotherapy and helps these tumours recur after treatment. We have discovered a new mechanism of chemotherapy resistance within the mitochondria that has the potential to be exploited to make cancer cells more sensitive to existing therapies.
Outsmarting the blood-brain barrier: A novel strategy for brain tumour treatment
Using gold nanoparticles, microbubbles and focused ultrasound we can localize chemotherapeutics to tumours in high concentrations. Tumour targeting antibodies attached to the gold nanoparticle allow glioma cells to internalize them, releasing their attached drugs within the tumour cell, resulting in cell death. Watch our animation demonstrating this process below.